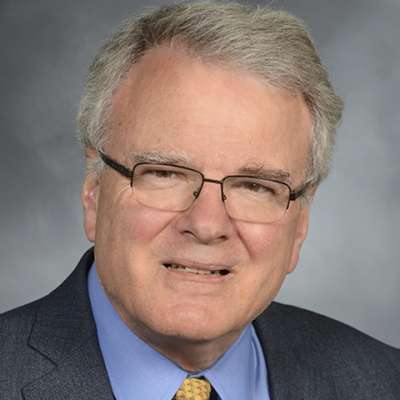
Olaf S. Andersen, M.D.
- Director, Weill Cornell/
Rockefeller/Sloan Kettering Tri-Institutional MD-PhD Program
1300 York Avenue, Room C-501 B
New York, NY 10065
Research Areas
Research Summary:
- Energetic coupling between membrane proteins and their host lipid bilayer
- Bilayer-mediated effects of biologically active molecules
- Bilayer-dependent basis for cytotoxicity
Membrane protein function varies with changes in the composition (and physical properties) of the bilayer in which the protein is embedded, and the bilayer properties may change not only in response to changes in lipid composition but also in response to drug partitioning into the bilayer. This regulation of protein function involves both specific interactions between the protein and individual molecules (drugs or lipids) in the bilayer, and more general interactions between the protein and the lipid bilayer as a liquid crystal with physical properties (thickness, intrinsic curvature and the associated elastic moduli).
This is important for two interrelated reasons. First, because the physico-chemical properties known to befall efficacious compounds — sufficient aqueous and lipid solubility — means that many, if not most, drugs are amphiphiles that partition into the bilayer/solution interface and thereby alter the bilayer’s physical properties. Second, because integral membrane proteins (e.g., receptors, channels and transporters) undergo conformational changes that involve the proteins’ bilayer-spanning domains, which are coupled to the bilayer core through hydrophobic interactions. The bilayer adaptation to a membrane protein’s hydrophobic domain, the protein-induced bilayer deformation, has an energetic cost \(\left( \Delta G_{def}\right)\) that varies with changes in protein shape and bilayer properties. The free energy difference of a conformational change between protein states I and II \(\left( \Delta G^{I\rightarrow II}_{total}\right)\) thus will be the sum of contributions from rearrangements within the protein \(\left( \Delta G^{I\rightarrow II}_{protein}\right)\) and within the bilayer \(\left( \Delta G^{I\rightarrow II}_{bilayer} = \Delta G^{II}_{def} – \Delta G^{I}_{def}\right)\). For integral membrane proteins, with their irregular protein/bilayer boundary, there will be an additional contribution from the inevitable residual exposure of hydrophobic and polar residues \(\left( \Delta G^{I\rightarrow II}_{res} = \Delta G^{II}_{res} – \Delta G^{I}_{res}\right)\).
An extensive literature has demonstrated the regulation of cell and membrane protein function by changes in lipid bilayer composition. This bilayer-mediated regulation of membrane protein function is important because changes in lipid bilayer properties, e.g. due to the partitioning of drugs into the bilayer/solution interface, will alter the \(\Delta G^{I\rightarrow II}_{bilayer}\) and \(\Delta G^{I\rightarrow II}_{res}\) contributions to \(\Delta G^{I\rightarrow II}_{total}\), providing a mechanism for the changes in protein function. When such drug-induced changes in the bilayer contributions to \(\Delta G^{I\rightarrow II}_{total}\) become sufficiently large they produce global effects and, eventually, cytotoxicity.
Current experiments address the following questions:
- What are the relation(s) between molecular structure and bilayer-modifying potency; can we predict the changes in bilayer properties (expressed as \(\Delta G^{I\rightarrow II}_{total}\)) based on a drug’s structure?
- What are the biological consequences of drugs bilayer-modifying effects; at what point do they become cytotoxic; can we predict if a drug candidate is likely to have undesired effects?
- What are bilayer-modifying effects of small peptides and proteins?
- Can we develop experimental strategies for distinguishing between specific and bilayer-mediated regulation of membrane protein function?
Recent Publications:
- Gautam, N, Elhusseiny, A, Mansour, M, Mehta, JL, Andersen, OS, Peng, Y et al.. Exploring the feasibility of using artificial intelligence to simulate the placebo arm of randomized clinical trials. Postgrad Med J. 2025; :. doi: 10.1093/postmj/qgaf095. PubMed PMID:40577492 .
- Ashrafuzzaman, M, Koeppe, RE 2nd, Andersen, OS. Intrinsic Lipid Curvature and Bilayer Elasticity as Regulators of Channel Function: A Comparative Single-Molecule Study. Int J Mol Sci. 2024;25 (5):. doi: 10.3390/ijms25052758. PubMed PMID:38474005 PubMed Central PMC10931550.
- Joyce, RL, Tibbs, GR, David Warren, J, Costa, CJ, Aromolaran, K, Lea Sanford, R et al.. Probucol is anti-hyperalgesic in a mouse peripheral nerve injury model of neuropathic pain. Neurobiol Pain. 2023;14 :100141. doi: 10.1016/j.ynpai.2023.100141. PubMed PMID:38099280 PubMed Central PMC10719523.
- Eastman, RT, Rusinova, R, Herold, KF, Huang, XP, Dranchak, P, Voss, TC et al.. Nonspecific membrane bilayer perturbations by ivermectin underlie SARS-CoV-2 in vitro activity. bioRxiv. 2023; :. doi: 10.1101/2023.10.23.563088. PubMed PMID:37961094 PubMed Central PMC10634736.
- Peyear, TA, Andersen, OS. Screening for bilayer-active and likely cytotoxic molecules reveals bilayer-mediated regulation of cell function. J Gen Physiol. 2023;155 (4):. doi: 10.1085/jgp.202213247. PubMed PMID:36763053 PubMed Central PMC9948646.
- Rüger, M, Seitz, AM, Nuss, K, von Rechenberg, B, Seitz, D, Kostmann, C et al.. A Bioinspired Orthopedic Biomaterial with Tunable Mechanical Properties Based on Sintered Titanium Fibers. Adv Healthc Mater. 2023;12 (2):e2202106. doi: 10.1002/adhm.202202106. PubMed PMID:36250334 .
- Bobe, K, Willbold, E, Haupt, M, Reebmann, M, Morgenthal, I, Andersen, O et al.. Biodegradable open-porous scaffolds made of sintered magnesium W4 and WZ21 short fibres show biocompatibility in vitro and in long-term in vivo evaluation. Acta Biomater. 2022;148 :389-404. doi: 10.1016/j.actbio.2022.06.005. PubMed PMID:35691561 .
- Cowan, LM, Strege, PR, Rusinova, R, Andersen, OS, Farrugia, G, Beyder, A et al.. Capsaicin as an amphipathic modulator of NaV1.5 mechanosensitivity. Channels (Austin). 2022;16 (1):9-26. doi: 10.1080/19336950.2022.2026015. PubMed PMID:35412435 PubMed Central PMC9009938.
- Maer, AM, Rusinova, R, Providence, LL, Ingólfsson, HI, Collingwood, SA, Lundbæk, JA et al.. Regulation of Gramicidin Channel Function Solely by Changes in Lipid Intrinsic Curvature. Front Physiol. 2022;13 :836789. doi: 10.3389/fphys.2022.836789. PubMed PMID:35350699 PubMed Central PMC8957996.
- Rusinova, R, He, C, Andersen, OS. Mechanisms underlying drug-mediated regulation of membrane protein function. Proc Natl Acad Sci U S A. 2021;118 (46):. doi: 10.1073/pnas.2113229118. PubMed PMID:34753824 PubMed Central PMC8609545.
- Kostmann, C, Lisec, T, Bodduluri, MT, Andersen, O. Automated Filling of Dry Micron-Sized Particles into Micro Mold Pattern within Planar Substrates for the Fabrication of Powder-Based 3D Microstructures. Micromachines (Basel). 2021;12 (10):. doi: 10.3390/mi12101176. PubMed PMID:34683227 PubMed Central PMC8540083.
- Christophers, B, Macedo, B, Nieblas-Bedolla, E, Marr, M, Andersen, OS, Boothroyd, C et al.. First-generation physician-scientists are under-represented and need better support. Nat Med. 2021;27 (5):752-755. doi: 10.1038/s41591-021-01352-3. PubMed PMID:33958798 PubMed Central PMC8211032.
- Ghovanloo, MR, Choudhury, K, Bandaru, TS, Fouda, MA, Rayani, K, Rusinova, R et al.. Cannabidiol inhibits the skeletal muscle Nav1.4 by blocking its pore and by altering membrane elasticity. J Gen Physiol. 2021;153 (5):. doi: 10.1085/jgp.202012701. PubMed PMID:33836525 PubMed Central PMC8042605.
- Sun, D, He, S, Bennett, WFD, Bilodeau, CL, Andersen, OS, Lightstone, FC et al.. Atomistic Characterization of Gramicidin Channel Formation. J Chem Theory Comput. 2021;17 (1):7-12. doi: 10.1021/acs.jctc.0c00989. PubMed PMID:33378617 PubMed Central PMC7808174.
- Sun, D, Peyear, TA, Bennett, WFD, Holcomb, M, He, S, Zhu, F et al.. Assessing the Perturbing Effects of Drugs on Lipid Bilayers Using Gramicidin Channel-Based In Silico and In Vitro Assays. J Med Chem. 2020;63 (20):11809-11818. doi: 10.1021/acs.jmedchem.0c00958. PubMed PMID:32945672 PubMed Central PMC7586341.
- Bosquesi, PL, Melchior, ACB, Pavan, AR, Lanaro, C, de Souza, CM, Rusinova, R et al.. Synthesis and evaluation of resveratrol derivatives as fetal hemoglobin inducers. Bioorg Chem. 2020;100 :103948. doi: 10.1016/j.bioorg.2020.103948. PubMed PMID:32450391 PubMed Central PMC8052979.
- Gotian, R, Andersen, OS. How perceptions of a successful physician-scientist varies with gender and academic rank: toward defining physician-scientist's success. BMC Med Educ. 2020;20 (1):50. doi: 10.1186/s12909-020-1960-9. PubMed PMID:32054479 PubMed Central PMC7020365.
- Sun, D, Peyear, TA, Bennett, WFD, Andersen, OS, Lightstone, FC, Ingólfsson, HI et al.. Molecular Mechanism for Gramicidin Dimerization and Dissociation in Bilayers of Different Thickness. Biophys J. 2019;117 (10):1831-1844. doi: 10.1016/j.bpj.2019.09.044. PubMed PMID:31676135 PubMed Central PMC7018991.
- Park, S, Yeom, MS, Andersen, OS, Pastor, RW, Im, W. Quantitative Characterization of Protein-Lipid Interactions by Free Energy Simulation between Binary Bilayers. J Chem Theory Comput. 2019;15 (11):6491-6503. doi: 10.1021/acs.jctc.9b00815. PubMed PMID:31560853 PubMed Central PMC7076909.
- Gutzeit, VA, Thibado, J, Stor, DS, Zhou, Z, Blanchard, SC, Andersen, OS et al.. Conformational dynamics between transmembrane domains and allosteric modulation of a metabotropic glutamate receptor. Elife. 2019;8 :. doi: 10.7554/eLife.45116. PubMed PMID:31172948 PubMed Central PMC6588349.